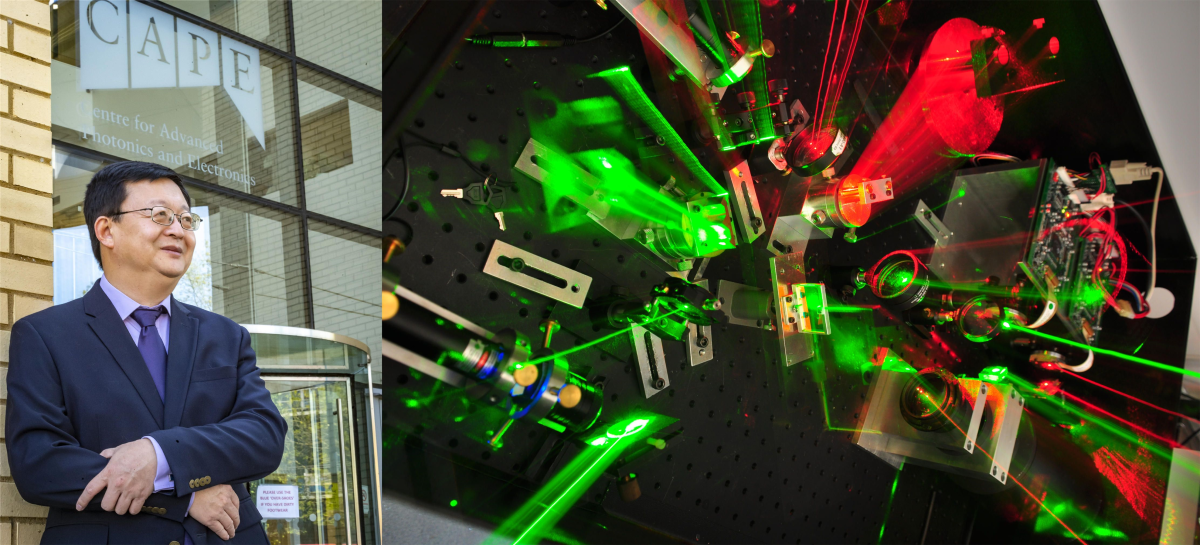
Submitted by Dr Mark Leadbeater on Mon, 09/08/2021 - 15:09
Many fields such as industrial manufacturing require 3D surface profile measurement with nanometre precision. Achieving this precision requires an instrument with incredibly high component accuracy. In this interview, Professor Daping Chu, described the project "Holographic Interferometer for 3D Surface" he is leading at the Cambridge University- Nanjing Centre of Technology and Innovation. Prof Chu explained why high precision 3D surface measurement is so difficult, and he described his solution and its advantages, and how it might be commercialized.
Professor Chu has been engaged in scientific research and commercialization for nearly 40 years, across a wide range of fields. With an academic background in physics, he has worked in applied research on non-volatile ferroelectrics, thin-film transistors, biosensors, liquid crystal, organic light-emitting, inkjet printing, additive manufacturing, 3D printing of complementary transistors and many other areas. His scientific research experience covers a wide range from developing materials and devices to commercializing products.
In the past ten years, Professor Chu's research has concentrated on optical holography and its applications. The research scope of optical holography is wide. His research not only covers the light source and the spatial light modulator, but also their application to reconstruct 3D display systems for use in trains and vehicle, or head mounted. His research also includes developing wavelength multiplexing and switching devices for all-optical communication networks. Many innovations have been successfully commercialized under his guidance, and several spin-out companies have been successfully acquired.
Professor Chu is currently a professor at the University of Cambridge, the director of the Centre for Advanced Photonics and Electronics and the director of the Centre for Photonic Devices and Sensors. Focusing on his research field of optical holography and spatial light modulation, the project is committed to achieving high-precision measurement of 3D surfaces.
Bottlenecks of 3D surface analysis with nanometre precision
Professor Chu described the aims of the project: when manufacturing components, conventional techniques give millimetre level precision, modern mechanical techniques give micron level precision, but nanometre precision is now needed: “it is increasingly urgent to search for convenient, efficient, and low-cost precision surface measurement methods."
Professor Chu described the two nanometre-level precision surface measurement methods currently used: contact and non-contact. He described the advantages and disadvantages of both.
The contact method uses a probe to measure surface height. For nanometre precision, an atomic force microscope is used. Though this method can be used under normal conditions, it has clear shortcomings: the probe will inevitably affect and damage the sample surface, and the area scanned is very small. The probe is extremely thin and so only measures a narrow range. Creating a two-dimensional 3D height map by scanning line by line is time-consuming, and the relationship between scanning speed and surface can cause human errors. Therefore, the probe method is impractical for high-precision measurement of large areas and large viewing angles.
The non-contact method has problems too. The surface morphology of the sample can be observed using a scanning electron microscope but it cannot measure the exact value of any surface fluctuation of the samples. Thus direct quantitative measurements is difficult.
Existing optical interferometers measure the surface profile by illuminating the surface with coherent light and studying interference patterns in the reflection. The nano-level morphology change of the sample surface is calculated from the phase difference arising from the different optical paths. However, Professor Chu pointed out that the non-contact method also has disadvantages:
First, the focused beam can only measure a small spot on the surface at a time and the field of view is small. Like the probe and scanning electron microscope methods, the sampling speed for the large sample surface is limited and the maximum height measured is usually only a few hundred microns.
Second, the optical path difference has two causes: the “true” undulating topography of the sample surface and effects from the unevenness of the optical lens and other optical system components. Distinguishing the two parts is a major technical challenge.
Objective: Realize 3D surface analysis with large field of view, large height difference and heterogeneous materials
So how can higher accuracy, faster measurement and larger field of view be achieved? Professor Chu’s project addresses these problems.
"This project introduces the holographic method and pure phase LCOS (liquid crystal on silicon) spatial light modulator into the traditional optical interferometer to comprehensively solve the problem of low efficiency and low precision", Professor Chu explained. First, sampling speed is much faster: the holographic method can simultaneously generate tens of thousands of light points, which combined with beam scanning can achieve large fields of view (centimetres, decimeters or even larger).
Second, the height of the LCOS spatial light modulator is adjustable, and the system phase difference can be corrected point by point to achieve precise calibration of the phase difference part of the spatial light modulator. The measured interference fringe information can compensate for the phase difference caused by the unevenness of the optical device hardware in the middle, and reduce the measurement error, which is difficult to achieve with the common measurement methods.
"The ability to solve the error caused by the periodicity of interference fringes and accurately measure the surface of heterogeneous material samples the two major technical contributions of this project", Professor Chu further explained, "These two have always been thorny problems in interferometer measurement. "
As far as the periodicity of interference fringes is concerned, the most critical element in phase interference is phase difference. When the optical path difference changes by one wavelength, the phase difference is 2π. If the height difference of the measured object surface exceeds one wavelength, it will cause the interference fringe to appear repeatedly due to the periodicity of the interference fringe. In other words, in the face of a large elevation difference surface morphology with a longitudinal measurement range exceeding the wavelength order, in addition to distinguishing the phase difference caused by the sample surface and the uneven optical components, we also need to correspond the repeated interference fringes to the optical path difference that produced it, so as to realize the seamless splicing and deduction of the overall interference fringes. The project is the first to introduce a spatial light modulator, which is expected to break through the constraints of high-precision surface analysis with large height differences.
Measuring the profile of devices made using several materials presents a particular challenge. For example, some devices will have semiconductors, dielectrics and metals on their surface. A homogeneous surface has constant reflectivity, and the reflection intensity and phase delay remain the same. This means that the phase difference is mainly due to the varying height of the surface. In this case, it is relatively easy to measure height precisely.
As different materials have different reflectivity, the phase difference comes from both the surface height difference and the surface materials restricting measurement accuracy. Current mainstream measurement methods seldom consider the influence of the material on the phase difference. The project is the first to use spatial light modulators to explore high-precision measurement of the surface of heterogeneous samples.
Encouraging commercialization: prospects for application by industry
A broad range of applications could use this new approach. At the macro-scale (several meters across), it can be used to measure the optical components of large astronomical telescopes. At the meso-scale (sub-millimetre), it can be used for processing of precision instruments such as precision lathe rotation shafts and engine parts. At the microscopic scale, the project could enable high-level etching of metamaterial structures such as integrated circuits, MEMS equipment and micro-nano devices. It could also be used for cell detection in biomedical applications.
The engineering and manufacturing advantages of the new approach are also significant. By replacing high-precision optical lenses and expensive piezoelectric driving devices with LCOS devices and holography, the weight and cost of the instrument are reduced. "The difference in core components means that the project needs to build an upstream and downstream industrial chain that is different from the traditional interferometer, which can form a complementary effect with the existing interferometer industry," Professor Chu further explained.
Professor Chu’s team has already overcome the limitations of LCOS devices used in display projectors. At the University of Cambridge, the team has a complete experimental line covering spatial light modulator development, ultra-clean assembly, operation, and high-speed hologram generation. It has developed a complete set of phase adjustment technology that achieved 6/8/10 bit phase difference measurement between 2π phases. The team’s LCOS product assembly technology is world’s leading, and an innovative company has been established to provide services to industry leaders; in Nanjing, both Dr. Deng Yuanbo, Co-PI of the project and postdoctoral fellow at Cambridge University, and local expert Professor Yang Haining from Southeast University, have rich engineering experience in optical measurement architecture. The Centre has already had a patent granted for "a fully automatic phase error correction method and system for holographic interferometers".
"Scientific research should be application-oriented, and technology should be used." Professor Chu finally emphasized that "the best sign of using technology is productization, marketability, and enabling users to benefit from new technologies." Focusing on the industrialization path of the project, he said that the project plans to develop the first-generation engineering prototype as the starting point, develop the "standard holographic interferometer" as the initial model for industrialization, and demonstrate the platform technology that has been formed to start innovative companies or carry out technology transfers. With one or two clear requirements as a breakthrough, it will develop corresponding testing instruments around specific application areas, extend different series of measurement products, and continuously optimize products according to market needs, so as to provide the ultimate measurement effect for different scenarios.
This interview with Prof Daping Chu originally appeared on the website of Cambridge University- Nanjing Centre of Technology and Innovation where Prof Chu is the Academic Director and CEO, a full version of the article can be read there.